Plant crops typically need supplemental nutrients. — AFP Relaxnews
France had unexpectedly imposed a strict Covid-19 nationwide lockdown last weekend, and this meant that our planned return home is not possible for the moment. So, I am scribbling this in the UK, whilst trying to establish a routine here conducive to my usual work and study habits.
I was walking around the farm fields here a few days ago and noticed some of the fields being treated with inorganic fertiliser. This got me interested in what chemicals make up industrial fertilisers and it is a more curious story than expected.
Limited time offer:
Just RM5 per month.
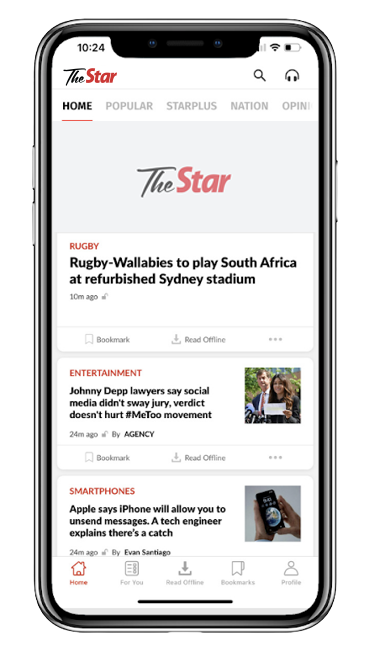